Treatment of Acinetobacter Infections: Understanding the Mechanisms of a Novel Podophage
Introduction
In recent years, bacteriophages have seen renewed interest as treatment for antibiotic resistant infections. One such hard to treat organism is Acinetobacter species, which are gram- negative bacilli prevalent in hospital settings, especially the intensive care unit, that can cause urinary tract infections, infective endocarditis, secondary meningitis, pneumonia and wound infections. Increased frequency of Acinetobacter infections, particularly Acinetobacter baumannii and Acinetobacter nosocomialis, presents a significant threat to humans. A large number of these strains are multi-drug resistant making treatment with traditional antimicrobial therapies more challenging. The ability to form capsules allows these bacteria to form biofilms that confer protection against host immune responses and antibiotics by protecting the outer membrane from the surrounding environment. This makes it particularly difficult to eliminate once established (4).
Phages can be used in whole (phage therapy) or in part (phage products) to clear bacterial infections, reduce cytopathic effects, or work synergistically with antibiotics. For phage therapy to be effective, the bacteriophages must be able to infect and neutralize the target bacterial cells (4). Lysogenic phages incorporate their genetic material into the bacteria in the form of prophages, which can cause it to become more or less virulent depending on the genes of the phage. These phages can be induced to become lytic phages either spontaneously or from bacterial cell stress (2). Lytic phages cause destruction of the bacterial cells they infect, releasing more phages in the process, which can aid in clearance. These phages are responsible for the breakdown of biofilms in nature and serve as a regulatory control of bacterial growth in the environment (3).
Finding and utilizing an appropriate phage for treatment of Acinetobacter infections is not without challenges. The presence of the biofilm can physically prevent phages from binding to receptors that are needed for penetration. The capsule, and subsequently the biofilm, is composed of exopolysaccharides (EPS) and are considered a virulence factor. Degradation of the EPS is necessary for effective treatment by both traditional antimicrobials and phages. The Petty phage was identified as a candidate capable of overcoming the EPS and selected for further analysis to understand the mechanisms utilized (1).
Hypothesis
Hernandez-Morales, et al, studied the novel podophage Petty to illuminate its potential as a therapeutic treatment for infections by A. baumannii and A. nosocomialis (1).
Methods and Results
The podophage Petty was isolated from sewage by researchers at Texas A&M, plated on A.nosocomialis and observed for plaque formation, depicted in figure 1. Plaques formed ~2 millimeter in diameter with a 25 minute latent period and 240 particle burst size. Adsoprtion of 90% of particles was seen within 10 minutes and the frequency of resistant cells was ~10-7 colony forming units. Transmission electron microscopy was used to determine virion structure, revealing a 60 nanometer head and 15 nanometer tail. After isolation, the host range of Petty was determined using a spot-dilution assay against 40 different strains of Acinetobacter, over half of which were multi-drug resistant. Plaques were observed in four strains of A. baumannii that had been isolated from patient cultures(1).
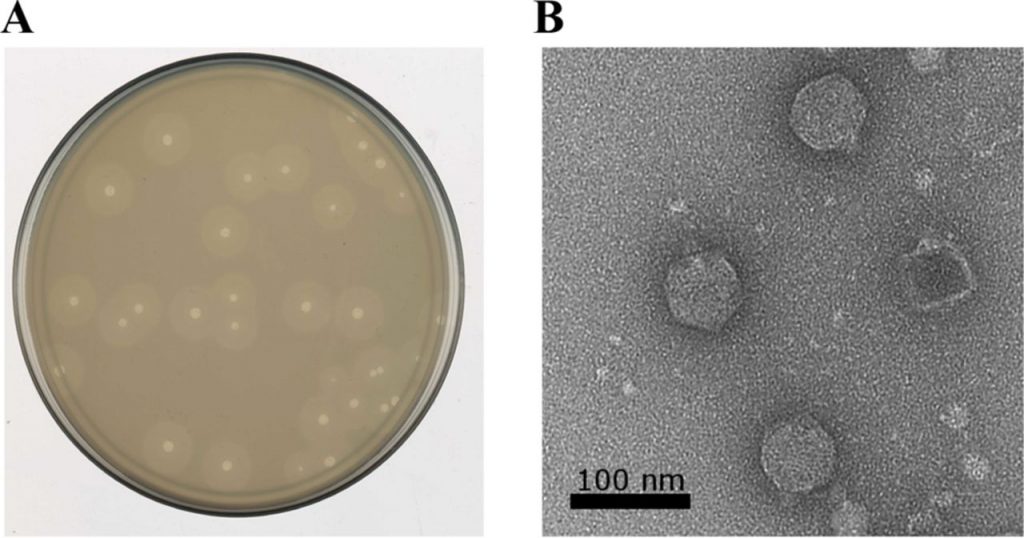
Further characterization of Petty was conducted through a genome analysis. It was found to code for 45 predicted proteins and 23 proteins of unknown function, with 11 unique proteins not seen in other phages. The genome was further compared to known phages to determine its phylogeny and mass spectrometry was used to identify its products. This enabled researchers to differentiate the novel aspects of Petty from known Acinetobacter phages to understand the mechanisms unique to this phage (1).
While Petty utilizes the classic holin-endolysin pathway to cause lysis. Holes are formed in the inner cell membrane by holin which allows exposure to the cytoplasmic endolysin that disrupts the cell wall. Based on a comparative analysis of these enzymes, researchers determined these genes are mosaic and likely evolve independently in phages with different host bacteria. To complete lysis of Gram-negative bacteria, phages utilizes spanins on the outer membrane. Neither of the two known spanins was found in the genome of the Petty phage, a trait shared among similar phages of Acinetobacter but different from similar phages that infect other bacterial species. The lack of spanins indicates two possible outcomes: incomplete lysis or explosive lysis. Petty was found to produce the latter, with explosive polar lysis observed via time lapse photographic analysis of pixel density, depicted in figure 2. This indicates Petty utilizes an alternative pathway. Subsequent analysis of the genome identified gp27 as a potential protein responsible for disruption of the outer membrane. This protein is unique to Petty, with no other gp27 homologs found in similar phages, but does share similarities to an autolysin from a Bacillus phage. It is also possible that the endolysin responsible for disruption of the inner membrane functions in the same way on the outer membrane leading to complete lysis (1).
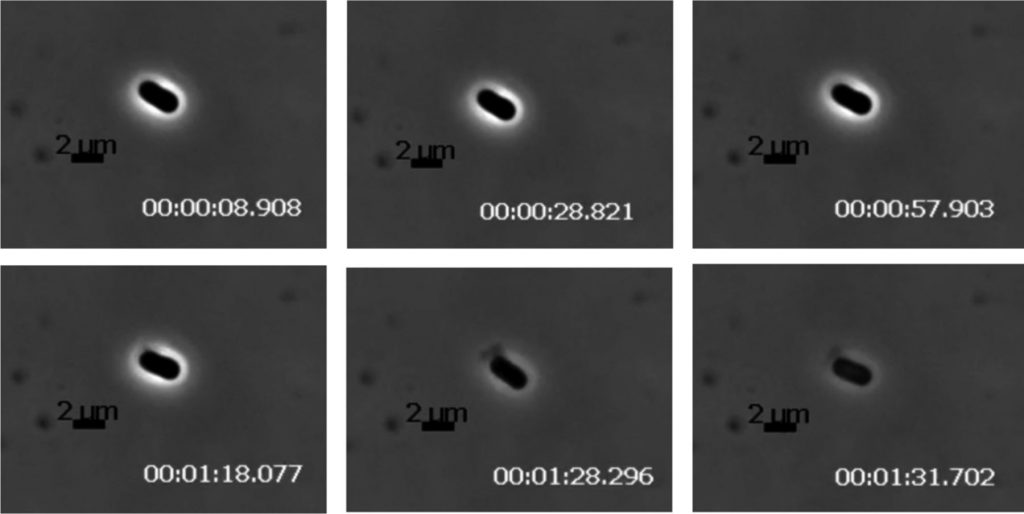
Crucial to the ability of Petty to infect Acinetobacter species is the disruption of the capsule to facilitate binding of the virus with receptors. This disruption was seen during plaque formation as an expanding halo around the infected cell. Researchers deduced this was due to depolymerase activity working on the polysaccharide capsule with the release of newly formed viral particles. Based on the location of depolymerase in other phages, researchers looked into the tail fiber genes and identified gene 39 as a likely candidate. This gene was cloned and inserted into a strain of Escherichia coli for amplification, purification and further analysis. The enzyme, named Dpo1, produced through recombination was of a similar molecular mass to that predicted based on the gene analysis. Application of Dpo1 to lawns of phage-susceptible Acinetobacter species produced translucent zones similar to those seen during plaque formation indicating the enzyme of interest had been properly identified (1).
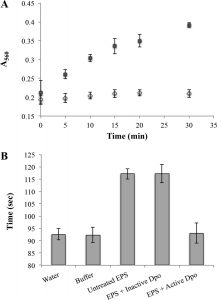
The activity of Dpo1 on the capsule was further studied in purified EPS. Active Dpo1 was found effective in reducing the viscosity of the purified EPS to one similar to water. Inactivated Dpo1 did not produce the same results, with the viscosity remaining similar to that untreated EPS, indicating the active enzyme is responsible for the changes in viscosity (see figure 3). Subsequent in vitro testing of the Dpo1 on Acinetobacter strains produced removal of approximately 20% of the biofilm. This indicates the activity of Dpo1 alone may not be sufficient to remove Acinetobacter cells (1).
Conclusions and Significance
Acinetobacter species are clinically significant due to the growing incidence of multi- drug resistant infections in immunocompromised patients. They account for an increasing number of nosocomial infections and have proven to be difficult to treat through traditional methods. The Petty phage has the potential to be a novel treatment option for these patients, with characteristics that make it uniquely capable of overcoming the traits that make Acinetobacter virulent (1).
The lysis mechanism of Petty is still not well understood, but is effective in creating explosive lysis without the use of spanins. This was found to be a common trait among similar Acinetobacter phages, but not to similar phages of other bacterial species. This may indicate there are unique characteristics to the Acinetobacter outer membrane that are disrupted by the known endolysin present (1). Additionally, there is the potential for the phage products to be utilized in conjunction with traditional treatments. Purified Dpo1 could be applied to the site of
Acinetobacter infection to remove the biofilm, thereby exposing the cells to antimicrobials and the host immune defenses.
Ethical issues
There are no ethical issues related to this study as all experiments were conducted using bacterial cells in vitro. The phage used was a lytic phage with little to no risk of confering additional virulence to the cells exposed. Further study of the Petty phage, either in situ or in vivo could raise ethical issues relating environmental impacts, treatment and care of animal models,
or informed consent in human subjects.
It is important to note that there is still a great deal to learn about phage replication cycles, both lytic and lysogenic. The high variability of both abiotic and biotic factors in nature may drive evolutionary changes to the way a phage operates that cannot be accounted for in vitro. Caution must be taken to avoid conferring virulence factors to bacteria through the use of phages as a therapeutic (3).
Subsequent testing
Further study of Petty is required to determine its efficacy in naturally-occurring Acinetobacter populations. An in situ analysis of the phage’s ability to clear Acinetobacter would provide additional support to its viability as a therapeutic treatment for infection. Laboratory experiments are conducted under ideal conditions, and are not necessarily representative of the outcomes that would be seen in the environment. Stress or nutrient scarcity may increase expression of capsule genes to avoid desiccation and could render the phage’s depolymerase activity ineffective. This would prevent binding of the phage to the bacteria and effectively block penetration. Studies have shown that phages utilizing depolymerase to degrade biofilms of multi- drug resistant bacteria with increased capsule expression. Confirmation of similar results with Petty would be appropriate before moving forward with subsequent experiments.
Following in situ studies, a safety study administering phages in the methods required for treatment of Acinetobacter infections to healthy, immunocompetent individuals would be necessary. Phages can be applied topically, orally, locally through injection, or intravenously. Each administration route would need to be studied to look for adverse effects and understand the host immune response to Petty specifically, including synergistic or deleterious impacts. This would need to be done in appropriate animal models before progressing to human trials, but will allow for a comprehensive analysis of the efficacy and adverse events of in vivo applications in the future. The initial results in combination with related studies of phage therapy show the potential for a promising alternative treatment for multi-drug resistant Acinetobacter infections.
References
(1) Hernandez-Morales, A.C., Lessor, L.L., Wood, T.L., Migl, D., Mijalis, E.M., Cahill, J., Russell, W.K., Young, R.F., Gill, J.J. (2018). Genomic and biochemical characterization of Acinetobacter podophage Petty reveals a novel lysis mechanism and tail-associated depolymerase activity. Journal of Virology, 92(6), 1-18. DOI: 10.1128/JVI.01064-17
(2) Howard-Varona, C., Hargreaves, K. R., Abedon, S. T., & Sullivan, M. B. (2017). Lysogeny in nature: Mechanisms, impact and ecology of temperate phages. The ISME journal, 11(7), 1511–1520. https://doi.org/10.1038/ismej.2017.16
(3) Lin, D., Koskella, B., & Lin, H. (2017). Phage therapy: An alternative to antibiotics in the age of multi-drug resistance. World Journal Of Gastrointestinal Pharmacology And Therapeutics, 8(3), 162. doi: 10.4292/wjgpt.v8.i3.162
(4) Tortora, G. J., Funke, B. R., and Case, C. L. (2013). Microbiology: An introduction (11th edition). Pearson.